New
Scientist, 14 november 1992
Mark Oliphant
dared to suggest that hydrogen could be an exploitable source of energy.
Sixty years on, he is still enthusiastic about its potential.
David
Ellyard
NOW in his 92nd
year, Mark Oliphant is wearing well. His legs may no longer carry
him with certainty; his hearing, impaired since childhood, may demand
that conversations be carried at above normal volume. But he still
stands tall. His ruddy face still routinely creases with mirth, aided
by the booming laugh that has been his trademark for so long.
It is his mind
that remains most active. Currently he is an evangelist for "hydrogen
power", the use of hydrogen, released from water by electrolysis or
some other process, as a fuel for vehicles or to power the innovative
high-temperature fuel cells being developed in Australia. The electricity
needed, he says, may well come from sunlight, captured by the high-efficiency
solar cells developed by Martin Green at the University of New South
Wales. Oliphant freely acknowledges the problems that remain, such
as storage. Yet he feels able to say with conviction: "I am confident
that such a system for producing power from hydrogen, the cleanest
of fuels, will prove itself early in the next century".
His interest
in hydrogen as a source of power is highly appropriate. His early
scientific reputation was built on hydrogen or, to be precise, on
pioneering researches he undertook into nuclear reactions involving
deuterium, a heavy istotope of hydrogen. There is a key difference,
of course. Today Oliphant sees hydrogen as a source of chemical energy
liberated when hydrogen is burnt in an engine or a fuel cell. Such
an energy source is within our grasp, with just some small matters
of technology or economics to be tidied up. In the 1930s, the promise
was something much more profound and much less accessible, energy
from within the nuclei of hydrogen atoms.
The story begins
in 1932 at the Cavendish Laboratory of the University of Cambridge.
The Cavendish was then at the height of its influence. Ernest Rutherford,
the New Zealand born physicist who had almost single-handedly created
the science of nuclear physics, had built a team of researchers who
led studies of the world within the atom. Some measure of the intellectual
resources of the Cavendish comes form the annual photograph taken
in 1932. Of the 30 or so staff and students gazing solemnly at the
camera, nine were already, or were to become, winners of Nobel prizes.
Oliphant stands in the second row of the group, fourth from the left
and almost but not quite behind Rutherford. It is a symbolic position.
Oliphant was not yet in the front rank of the Cavendish, but he was
not far behind.
By the start
of 1932, he had been at the Cavendish a little over four years. He
had his doctorate and his first research papers in print. He was also
growing close to Rutherford, professionally and personally. They were
both antipodeans, given to plain talking. Oliphant and his wife Rosa
were often guests of Ernest and Mary Rutherford at their holiday cottage
in the north of Wales. And Rutherford appreciated Oliphant's growing
reputation as a designer, builder and operator of the complex apparatus
on which progress in nuclear physics was increasingly depending.
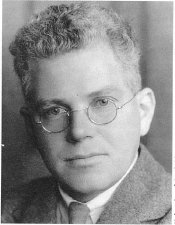 |
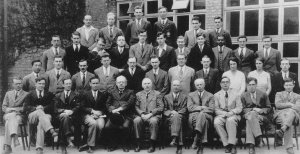 |
Arguably 1932
was the year of greatest achievement at the Cavendish with three discoveries
of such importance that they truly deserve the overworked accolade
"breakthrough". For the previous two decades, the standard model of
the atom had contained two types of particles: the light, negatively
charged electrons and lumps of positively charged matter called protons.
Then in the space of a few months, the number of known fundamental
particles doubled from two to four; the atoms of the lighter elements,
far from being indivisible, were broken open at will; and powerful
new machinery for both producing and detecting the particles came
into use. These discoveries gave nuclear physics a new impulse. And
the four men most closely involved at the Cavendish became Nobel laureates.
First to shine
was James Chadwick, Rutherford's greatly respected second-in-command.
At the beginning of 1932, he followed up an old idea of Rutherford's
and some recent discoveries in France and Germany, and with brilliant
insight identified a third fundamental particle- the neutron, close
to the proton in mass but with no electrical charge.
John Cockcroft
and Ernest Walton came next when, in April, they "split the atom".
They had built one of the first particle accelerators, a sort of gun
which used electrical potentials of several hundred thousand volts
to send protons (the nuclei of hydrogen) speeding down an evacuated
tube to collide with targets made of light elements such as boron
and lithium. A multitude of tiny flashes on a screen covered with
zinc sulphide revealed that, under proton bombardment, such light
elements disintegrated into alpha particles, the nuclei of helium
atoms.
Patrick Blackett
completed the run of successes in August when he developed an improved
version of the cloud chamber (another Cavendish invention) into a
powerful research tool for revealing the paths of the particles in
cosmic rays. With this, he trapped evidence of a new form of matter.
This was the positron, identical to the electron except that it was
positively charged; it was the first evidence of "antimatter".
Oliphant was
only a spectator of these triumphs. His own research interests lay
elsewhere. That was soon to change. Shortly after Cockcroft and Walton
split the atom, the Rutherfords invited the Oliphants to their Welsh
retreat. Conversation turned quickly to the recent breakthrough. Rutherford
had never been overly keen on large and complex apparatus, fearful
that building and running such machines might take precedence over
what really mattered, the collection of experimental data. Yet he
saw that times were changing and proposed a joint project that would
explore where Crockcroft and Walton's work was pointing. The first
task was to design and build an accelerator of the Crockcroft-Walton
type, but with some new features.
Room
with a past
Oliphant went
to work in the summer of 1932 in a couple of low-ceilinged, stone
flagged rooms in a historic precinct of the Cavendish basement. In
one of the rooms, Lord Rayleigh, an early director of the Cavendish,
had made the first precise measurements of the value of the ohm, the
unit of electrical resistance. In the same room, Rutherford and Chadwick
all but achieved the dream of the alchemists in 1919 when they transmuted
matter for the first time, turning atoms of oxygen into nitrogen.
Oliphant's accelerator
was as similar to Cockcroft and Walton's as a machine gun is to a
cannon. Their accelerator produced a beam of very powerful protons,
carrying up to 600 000 electronvolts of energy. Oliphant's machine
yielded protons carrying at most 200 000 electronvolts, but delivered
a hundred times as many protons to the target, so greatly increasing
the chances of a nuclear reaction taking place. With counting chambers
and electronic amplifiers replacing the eye and the sulphide screen,
debris flying from the point of impact could be measured more precisely
Rutherford was
very busy, with a laboratory to run and commitments that regularly
took him out of town. This meant he kept his colleague on a very long
leash, though the great man would drop in on Oliphant and his co-workers
once or twice a day if he could, to see how things were going. He
was intensely interested in progress, and his brilliant insights and
explanations of observations were beyond price. But Oliphant really
ran the show. His name was to come first on the half-dozen scientific
papers hewn from the mountain of data yielded by the basement accelerator.
Rutherford's name came last, if it appeared at all.
For the first
few months, Oliphant and Rutherford used beams of protons to break
open the nuclei of lithium, boron and beryllium, as Cockcroft and
Walton had done, though with greater precision. By so doing they cleaned
up many of the small uncertainties and turned out a paper or two.
The project
took a leap forward in the summer of 1933, with a visit by Gilbert
Lewis, a chemist from the University of California at Berkeley. Lewis
had a present for Rutherford, a few drops of the newly isolated "heavy
water". In this precious liquid, the hydrogen in many of the water
molecules had been replaced by "heavy hydrogen", twice as heavy as
the more common form. Although the Americans had already named the
new form of hydrogen "deuterium", and its nucleus "deuton" (now changed
to deuteron), Rutherford obstinately insisted on calling the atom
"diplogen" and the nucleus "diplon". In the wake of Chadwick's discovery
of the previous year, it was clear that each diplon contained a proton
and a neutron. The diplons made most effective bullets for the Oliphant
gun, producing many more transmutations than the single proton of
a hydrogen nucleus.
Soon, however
confusion set in. Oliphant tried a number of elements as targets,
but the results all looked much the same. The debris clattering into
the counting chamber was almost always dominated by protons with a
constant energy-enough to travel 14 centimetres in air before coming
to rest. There was some evidence that neutrons were present, although
these were difficult to detect. Similar work was going on in Berkeley
led by Ernest Lawrence, then a rising star in nuclear physics. The
Berkeley researchers had an explanation, the deuton (sticking to their
nomenclature) was an unstable particle which on impact with the target
shattered into a proton and a neutron.
Sticking
to the target
Not so, said
Oliphant. He suspected that the answer lay with contamination of the
target, because he had observed that the number of protons increased
with time. If the diplons fired from the beam somehow stuck to the
target, they would themselves become targets for more diplons coming
behind. Oliphant (and Cockcroft, who was loading his accelerator with
the same stuff), already knew that cleaning an exposed target greatly
reduced the number of protons.
To settle the
matter (and the well-mannered argument between Cambridge and Berkeley),
Oliphant had a colleague make targets from ammonium sulphate and phosphoric
acid, replacing some of the hydrogen in these compounds with heavy
hydrogen. There was no doubt about the result. The characteristic
pattern of protons appeared at once. Clearly the real collisions were
between diplons, rather than between diplons and the original target
material. Lawrence was quick to agree, with good grace, that Oliphant
had been right.
The clash of
the diplons still posed some puzzles. The matter become even more
complex when Oliphant went hunting for any other particles that might
be produced by the impact of diplon on diplon. His detection apparatus
included a sheet of mica to determine the energy of particles leaving
the site of collisions. These were of different thicknesses to represent
the stopping power of various thicknesses of air. With the help of
Rutherford's technical assistant George Crowe, Oliphant split a sheet
of mica so thin that it showed dazzling interference colours. With
that ultrathin film, they were able to observe a second group of particles,
roughly equal in number to the protons, which only had enough energy
to travel 1.6 centimetres through the air before coming to rest. These
particles carried a single charge, and so were still hydrogen nuclei.
But their tiny range indicated they must be heavy: the mass/energy
calculations showed that they weighed three times as much as ordinary
hydrogen. It could only be a hydrogen particle of mass 3.
The basement
accelerator had delivered its first unique discovery Rutherford and
Oliphant, claiming the right that falls to explorers of new territory
called the particles tritons and the element tritium. The family of
hydrogen nuclei, until recently consisting of one member, now had
three members.
There remained
yet another puzzle-that of the lone neutron. Clearly a pair of diplons
contributed two protons and two neutrons to each interaction. For
an instant, a particle with two protons and two neutrons must have
existed, the result of the fusing of the two diplons. In the observed
outcome, one proton emerged alone, and the other proton clumped with
the two neutrons to make a triton. But there was also a neutron that
emerged alone. Presumably this lonely neutron left behind two protons
and the other neutron, hanging together in a particle with two units
of charge and three units of mass-a helium nucleus of mass 3.
Discovery
of a lifetime
It was the most
important discovery of Oliphant's career. Of course the existence
of helium-3 was not demonstrated, merely inferred, on the ground that
it was the best explanation. The helium-3 left the target with such
meagre energy-equivalent to a range of 0.6 centimetres
in air-that it defied detection for another two years.
It is not uncommon
for major discoveries like these to develop a mythology that can obscure
the historical details. Oliphant has for many years told a story about
Rutherford and helium-3, an anecdote full of vivid detail. After many
hours puzzling over the data, the story goes, Oliphant went home dispirited
at the lack of progress. He was awoken in the small hours by a phone
call from Rutherford, who boomed that he knew the identity of the
short-range particles which had been seen. They were helium particles
of mass 3. Oliphant, taken aback by the suggestion, gently asked what
reasoning lay behind it. The phone shook as Rutherford roared back:
"Reasons? Reasons, Oliphant? I don't need reasons. I feel it in my
water!"
Of course the
short-range helium particles of mass 3 had not yet been seen, but
the facts do not really matter here. The story is more valuable for
what it says about the Rutherford style, the way his mind worked and
his impact on those around him. Such was the Rutherford spell that
even Oliphant, who was now one of those closest to him , was loath
to do anything of which Rutherford might disapprove.
Such caution
caused Oliphant to wait until Rutherford was away before embarking
on a secret investigation. Given that energy was released when diplons
fused together, could more energy be extracted than was needed to
bring the particles together, could such nuclear fusion be a net source
of energy? With the help of Crowe, Oliphant lashed together equipment
which fired a beam of accelerated diplons into a tube filled with
heavy hydrogen gas. This pioneering experiment came nowhere near succeeding
as there was far too little energy to begin with. But even the attempt
was enough to arouse Rutherford's ire when he heard about it. Rutherford
was adamant that atomic nuclei could never produce useful energy,
calling the notion "moonshine". Within a decade he was to be proved
wrong, with the first uranium fission reactor going critical in 1942.
In the case
of hydrogen fusion, progress has been much slower, and useful power
from fusion reactors is still decades away, if and when the day comes,
Mark Oliphant will have a share in it, at least in spirit. It was
he who first defined the vital reactions between deuterium particles,
and first dared to suggest that those reactions offered a promise
of power.
A
life of building particle accelerators for others
MARK OLIPHANT
was never again to be as productive in research as he had been in
those few years at the Cavendish in the 1930s. Thereafter, he was
to be a builder of particle accelerators for others to use.
His success
with the deuterium reactions, combined with Rutherford's patronage,
brought him recognition in the form of an FRS (he is one of the few
to have been a fellow for more than half a century). In 1935 he took
over Chadwick's old job as deputy director, and oversaw the construction
of two more particle accelerators.
But he was now
an attractive candidate for a "show of his own", and in 1937 the University
of Birmingham offered him a chair in physics. It was a timely move
for Oliphant. The influence of the Cavendish in nuclear physics was
waning with the departure of Blackert, Chadwick and others, and Rutherford
died around the time of Oliphant's departure. At Birmingham, he quickly
secured a massive grant to build the biggest cyclotron in Europe.
The war years
saw his influence grow though his ebullience and openness were seen
by some as an inappropriate lack of discretion. The magnetron, which
turned radar into a war-winning weapon for Britain, was invented and
refined in his Birmingham laboratories. In spring 1940, he was able
to bring the famous Frisch-Peierls memorandum to the attention of
the authorities. Written by two German émigré physicists
Otto Frisch and Rudolf Peierls, the memorandum told of the possibility
of an atomic bomb, and stimulated the formation of the Maud committee
and the quest for the atomic bomb.
Oliphant's friendship
with Ernest Lawrence, begun in scholarly dissent, strengthened in
the war years. In 1941, they shook the American scientific establishment
out of its indifference to the military potential of the recent discoveries
in nuclear physics. Once the Manhattan Project was under way Oliphant
moved his team to Berkeley to work with Lawrence on the electromagnetic
separation of the isotopes of uranium. After the war, shocked by the
carnage of Hiroshima, Oliphant became an evangelist for the "peaceful
atom", though his enthusiasm waned in later years.
In 1950, he
returned to Australia to become the first director of the Research
School of Physical Sciences at Canberra's Australian National University,
which he helped to found. He was the only founding father to put his
own career on the line by taking up a position at the university.
The Canberra years mixed triumph with disappointment. Under his leadership,
the school gained an international reputation. He was instrumental
in setting up the Australian Academy of Science in 1954, and served
as its first president. But his ambitious endeavour to build a massive
synchrotron ended in failure. His critics dubbed it "the white Oliphant";
Oliphant retired
in 1962, but in his early seventies he served five years as state
governor of south Australia, bringing his own style to that office.
David Ellyard
is a commentator on science and technology appearing on Australian
radio and television. He is co-author with Stewart Cockburn of The
Life and Times of Sir Mark Oliphant (Axiom Books, Adelaide, 1981).
Back to top